(Quantum) Sensing the Unknown
How quantum sensors could lead to the next Google Maps... Or to nuclear war
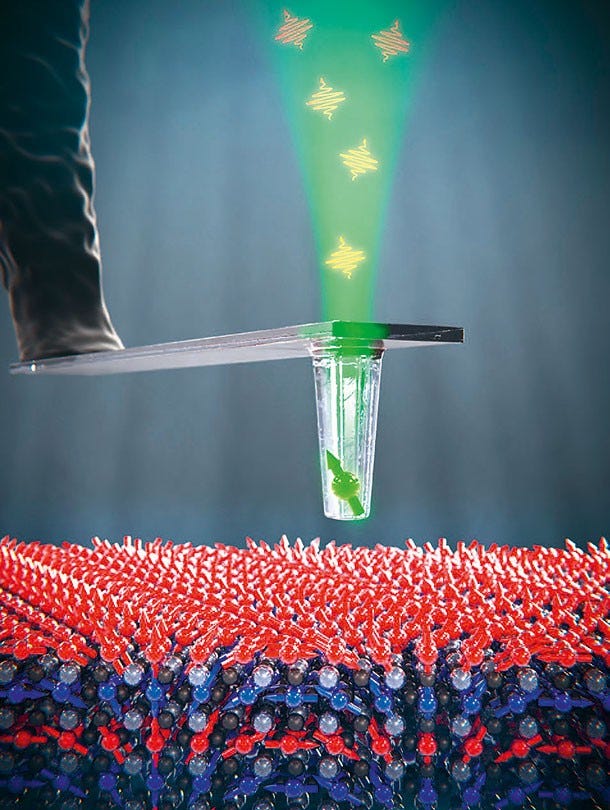
Welcome to Space Race, where we explore the impact of breakthroughs in science research on the intensifying global contest of superpowers in the 21st century. Subscribe for weekly breakdowns of the scientific breakthroughs that will shape the coming century.
In today’s newsletter, we look at quantum sensing and how it’s letting us learn about our environment right down to the atomic level. We’ll explore two types of widespread quantum sensors, the SQUID and atom interferometer. Finally, we’ll look at some applications, from mapping the underground to hunting nuclear submarines.
Richard Feynman, one of the most prolific physicists of the 20th century, famously said that “nobody understands quantum mechanics”. While physicists around the world still spend ample time pondering its consequences, quantum has already long become an indispensable part of our technological stack. Devices such as the transistors and semiconductors that power our computers are examples of the first generation of quantum technology, dating from the late 20th century. In the 21st century, we are in the midst of a second quantum revolution powered by innovation from corporate giants such as Google, Microsoft, and Amazon and billions in funding from global heavyweights such as the US, China, and the European Union. As the global technological competition heats up, one of the most mature technologies of this second revolution that is poised for breakout applications is quantum sensing.
What is Quantum Sensing?
One of the most fundamental applications of technology is to learn more about the environment around us. From thermometers to metal detectors, there are plenty of examples of devices that give us information about our environment. With these tools at our disposal, we can make informed decisions. If the temperature outside is hot, we will wear a t-shirt instead of a jacket. If Ancient Egyptians saw that the Nile was going to flood thanks to their trusty nilometer, they could take action to protect their fields.
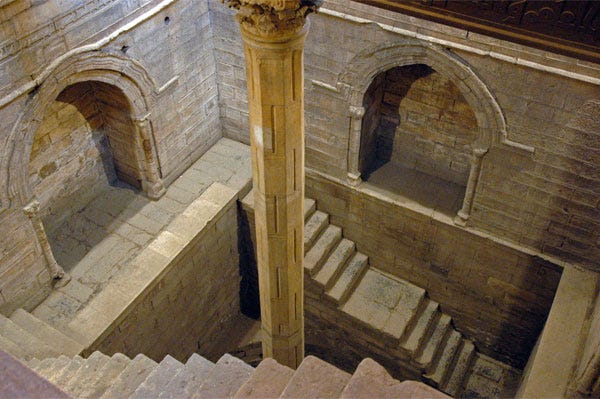
From the nilometer to the present, sensing instruments have been with us for thousands of years. As science advanced, our sensing instruments became more powerful and able to detect ever finer details about our environment. However, they couldn’t quite reach the smallest scales of nature, because despite our scientific progress we never had a truly accurate theory of nature at the atomic level. This changed with the arrival of quantum mechanics.
Quantum mechanics describes the properties of nature at the smallest scales right down to the atomic nucleus. For some perspective on how small this is, you could fit about 1,000,000,000 nuclei across the width of a single tiny human hair. Despite these minuscule (microscopic is too large an adjective!) length scales, quantum theory has been rigorously tested and confirmed to exquisite precision.
For the first time in history, we are armed with an understanding of how nature really works at the smallest levels and we can now put this knowledge to use in probing our environment. The addition of quantum mechanics to our millennia long quest to learn about our surroundings has led to the emergence of quantum sensing.
What are Current Quantum Sensors Like?
The best way to get a feel for quantum sensors is to learn about some real world examples. Two examples which illustrate the powerful capabilities of current quantum sensors are the Superconducting Quantum Interference Device — AKA the SQUID — and the Atom Interferometer.
SQUIDs
The wonderfully named SQUID is a quantum sensor that is capable of measuring extremely small magnetic fields. Basic SQUIDs first appeared in the 1960s following work at Ford and Bell Labs and since then have undergone considerable improvements. Today, a state of the art SQUID can measure magnetic fields 10,000,000,000,000 times smaller than the Earth’s magnetic field.
As their name suggests, SQUIDs were not possible before quantum mechanics since they rely on intrinsically quantum effects to achieve their phenomenal precision. One such effect is superconductivity - the remarkable fact that at extremely cold temperatures some metals stop being resistive and allow currents to flow forever. Superconductivity was discovered by Dutch physicist Heike Kamerlingh Onnes in 1911, and was explained in terms of quantum mechanics by Bardeen, Cooper, and Schrieffer only in 1957.
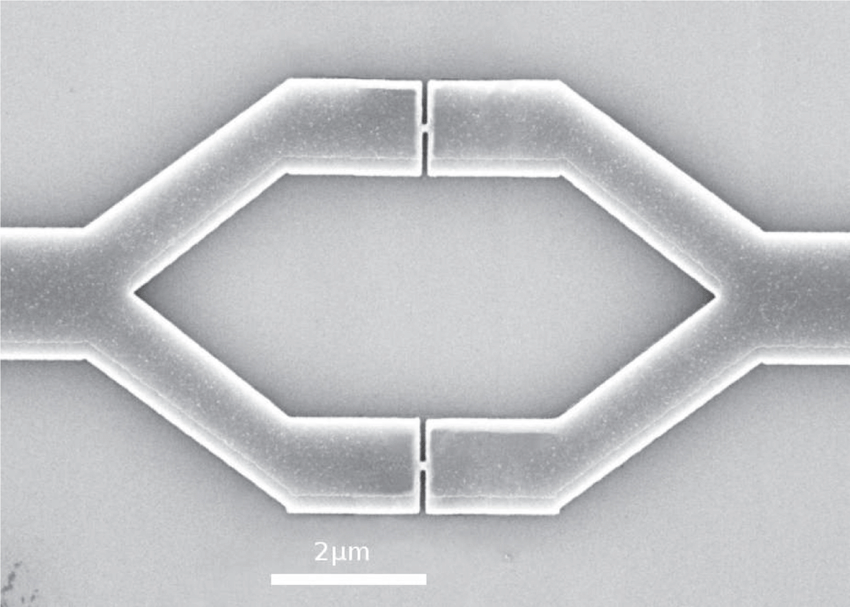
Ignoring a lot of nuance, the basic idea of a SQUID is a superconducting loop enhanced with a quantum device called a Josephson Junction. When a magnetic field goes through the loop, the voltage and current across the SQUID change and these changes can be measured. Since a SQUID is a fundamentally quantum device, extremely small quantum scale changes in the magnetic field will cause readily observed changes in the electrical behaviour of the SQUID. This sensitivity is what allows the SQUID to measure such small magnetic fields.
Atom Interferometers
While SQUIDs rely on superconductivity to measure magnetic fields, atom interferometers measure gravitational fields by relying on another profoundly bizarre property of quantum mechanics — the idea that everything is a wave and a particle at the same time.
Let’s pause for a second to consider what this wave-particle argument actually means. When we say particle, we imagine something like a very small billiard ball. If you fire two balls at each other, they will crash off one another and fly off in opposite directions. When we say wave, picture waves on the surface of a pond:
When two waves are directed at each other, they don’t bounce off each other like billiard balls. At points on the path where wavefronts from the two waves touch, we instead observe interference — the two waves either add or subtract to give a new pattern at those locations. In the video below, two wave sources emit waves which give an interference pattern in the middle where two waves meet.
Historically, we believed that wave and particle were two fundamentally different pictures. Atoms were particles because we could fire them at each other and see them bounce around, while light was a wave because it would make beautifully intricate interference patterns. Quantum mechanics threw all this on its head by instead showing us that everything is both wave and particle at the same time. This is known as wave-particle duality.
Wave-particle duality is the fundamental quantum effect that atom interferometers use to measure gravity. As the name suggests, it is the atoms that interfere with each other because they also behave like waves. You might ask why can’t we just use light instead of having to rely on this quantum weirdness with atoms. The answer is that gravity doesn’t affect light at all, but it does affect atoms. If we didn’t have quantum weirdness to help us we’d have no way hope of measuring any type of gravitational interaction at such small atomic scales.
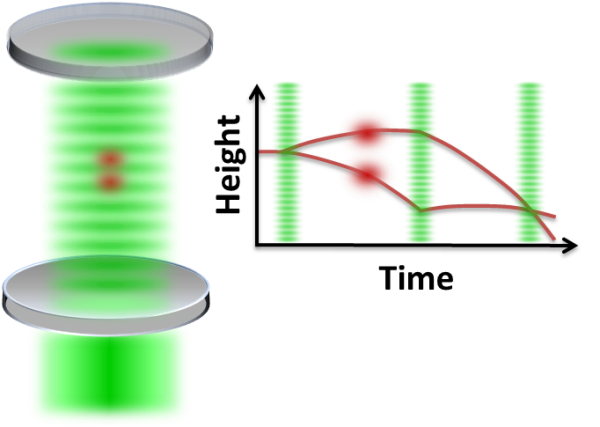
Once we accept that atoms are also wave-like and can interfere, the basic idea of an atom interferometer is straightforward. A beam of atoms in different quantum states is dropped down a height. The different types of atoms will interact slightly differently with gravity as they fall. Due to this difference, when they meet at the end these atoms will interfere with each other (thanks to their wave-like nature) and produce an interference pattern. By measuring the interference pattern, we can calculate the strength of the gravitational force which made the atoms fall.
As SQUIDs did for magnetic fields, atom interferometers have measured gravitational fields to exquisite precision. One great recent example is from 2019, when a team from Berkeley measured gravitational strength to 100,000,000 times smaller than the Earth’s gravitational field by driving an atom interferometer in a truck around the Berkeley hills.
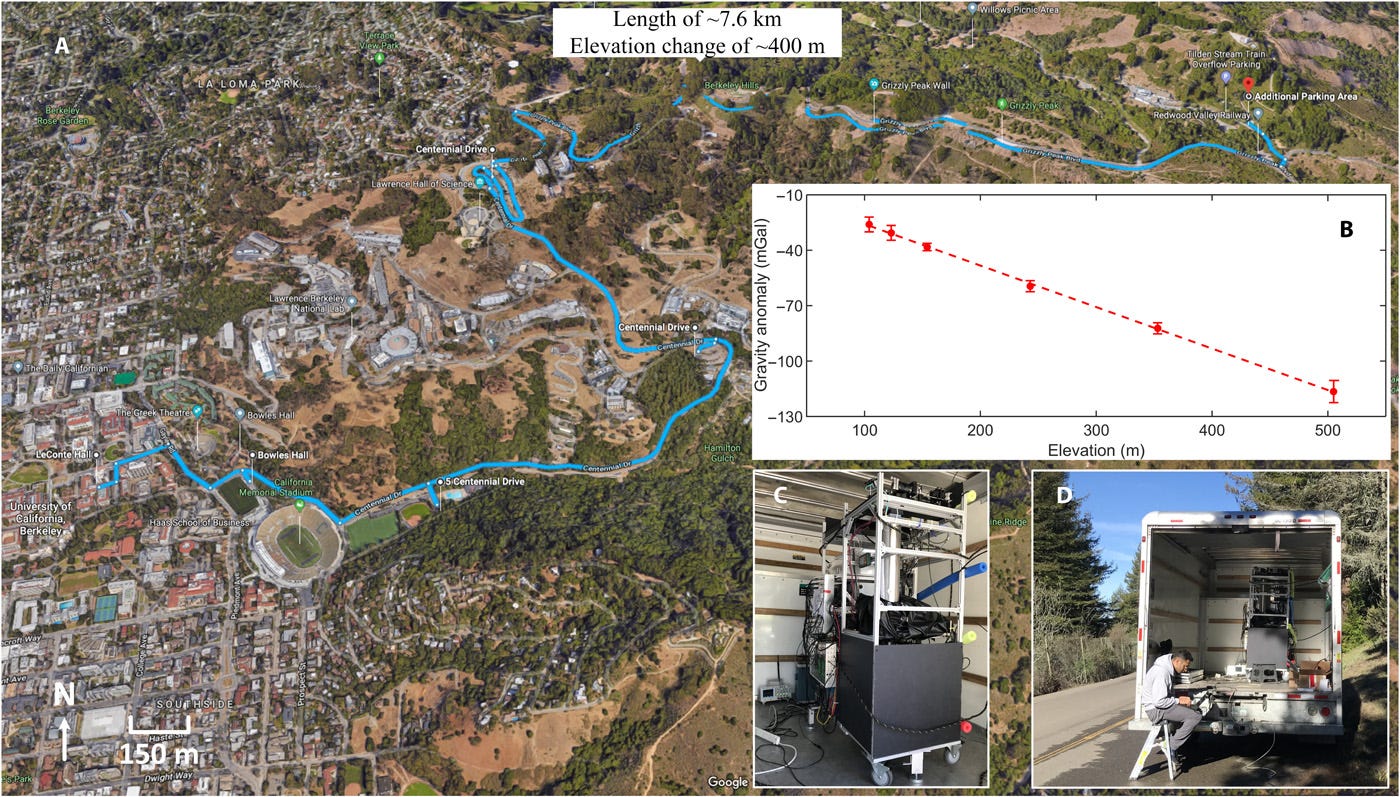
Perhaps this truck could be the proto version of a future quantum Google Maps car mapping the underground as it drives along?
The Big Picture of Quantum Sensing
The ability to sense the environment to new levels of precision already has numerous impacts beyond the lab. In addition to many possible commercial uses, the US Department of Defense has already identified quantum sensing as a “mature” application of quantum technology that is “poised for mission use” in militaries.
Sinkholes, Submarines, and the South China Sea
The atom interferometer gravimeter that we have discussed has great potential beyond basic science. As it is sensitive to such small changes in gravitational field, a quantum gravimeter could detect underground features such as mineshafts, sinkholes, pipes, cables and other utility infrastructure whose impact on the Earth’s gravitational field would otherwise be entirely undetectable. If the underground is more clearly mapped, building design can be improved to mitigate any risks that might be present underground. They could also detect natural features such as deposits of minerals and natural gas. Many private sector companies, such as Atomionics in Singapore, have already moved to try capitalise on these possibilities.
If they could see sinkholes and pipelines, quantum sensors could also detect large underground structures such as hidden nuclear silos. China is reported to have a vast network of underground tunnels to transport its Intercontinental Ballistic Missiles (ICBMs), but due to the great secrecy around them very little is known of their operational nature. Atom interferometry could allow mapping of this ICBM transport network, or of the many underground nuclear silos in the US instead.
Pause on that possibility for a second. Mapping an adversary’s nuclear assets is tremendously valuable intelligence. The only real deterrent to rogue nuclear powers is their adversaries’ ability to retaliate with their own nuclear strike. If that rogue power now knows where its target’s nuclear weapons are, it can launch a first strike to destroy them and eliminate the nuclear deterrent. If this were to happen, we would enter a much more dangerous world where the possibility of mutually assured destruction might not ward off nuclear warfare.
To be sure, quantum sensors can’t perform to this level currently, and this remains only a possibility for now. Nevertheless, it is a very real possibility and its consequences are worth thinking about. If one state somehow gets the capacity to pinpoint nuclear weapons through quantum sensing, it would be in the global interest for that technology to proliferate rapidly. This way, all states could map each others’ nuclear arsenals and this could restore the equilibrium of mutually assured destruction. From a more optimistic lens, if quantum sensors could identify nuclear assets it could also be a huge boost to non-proliferation efforts as countries such as Iran and North Korea would have difficulty hiding their nuclear programs.
The strategists will also point out rightly that many countries possess a nuclear triad — the ability to launch nuclear weapons from not only land but also air and sea. Atom interferometry could only really be effective for the land leg of that triad, so if the other legs remain unaffected, then mutually assured destruction has a better chance of remaining effective.
Except quantum sensing might have to say something about those other legs also — more specifically about nuclear submarines. For a long time, these have been extremely difficult to track due to the vastness of the oceans and submarine stealth technology. While normal submarines have to surface from time to time to refuel, nuclear submarines can remain submerged for months and evade detection thanks to their nuclear reactor power generators.
Many countries have experimented with the possibility of using SQUIDs to detect nuclear submarines. This would work by detecting the tiny magnetic ripples they cause as they move through the oceans, but these attempts have been mostly unsuccessful since SQUIDs are too sensitive and and get overwhelmed by even small disturbances in a noisy environment like the ocean.
However, in 2016 a team from China claimed that they could use an array of multiple SQUIDs together to detect the tiny disturbances of the Earth’s magnetic field caused by a submarine. The idea is that the data from the various SQUIDs could be used to average out the noisy unwanted information, but this claim still remains contested. If it is true, it will take on a much sharper importance given the recent agreement between the US, UK, and Australia to build the Australian Navy’s first ever nuclear submarines, which could prowl sensitive waters such as the South China Sea.
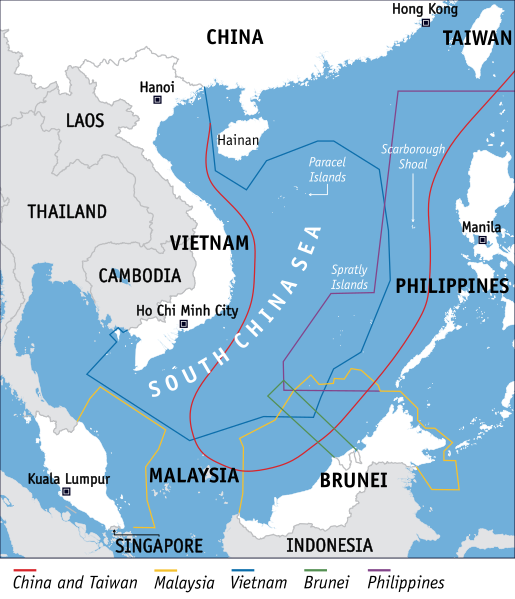
As the competition between the US and China intensifies, we could see quantum sensors swaying the strategic balance of power between the two superpowers in arenas like the South China Sea and beyond.
GPS
A less belligerent but equally exciting application for quantum sensing is GPS. Current GPS systems work by communicating with satellites, however they become ineffective if this link is broken. That could happen anywhere the GPS receiver cannot talk with enough satellites, such as a dense forest or a city full of skyscrapers. It could also happen if a malicious third party deliberately interferes with the communication.
However, it is much more difficult to tamper with the Earth’s magnetic field. By using a very sensitive magnetometer, one could measure this magnetic field and use it as a sort of “magnetic map” to track location. Designs for such new magnetic GPS systems already exist, but instead of SQUIDs they use another quantum technology called an NV centre. These are special types of diamonds with certain atoms missing in the crystal lattice — the NV stands for “nitrogen vacancy”. If we shine light of a particular frequency at the NV centre, it will emit a different frequency of light which depends on the magnetic field, allowing us to measure the field at the location of the NV centre. This technology would be a little less accurate than GPS, but it would be more reliable and robust against threats like external jamming.
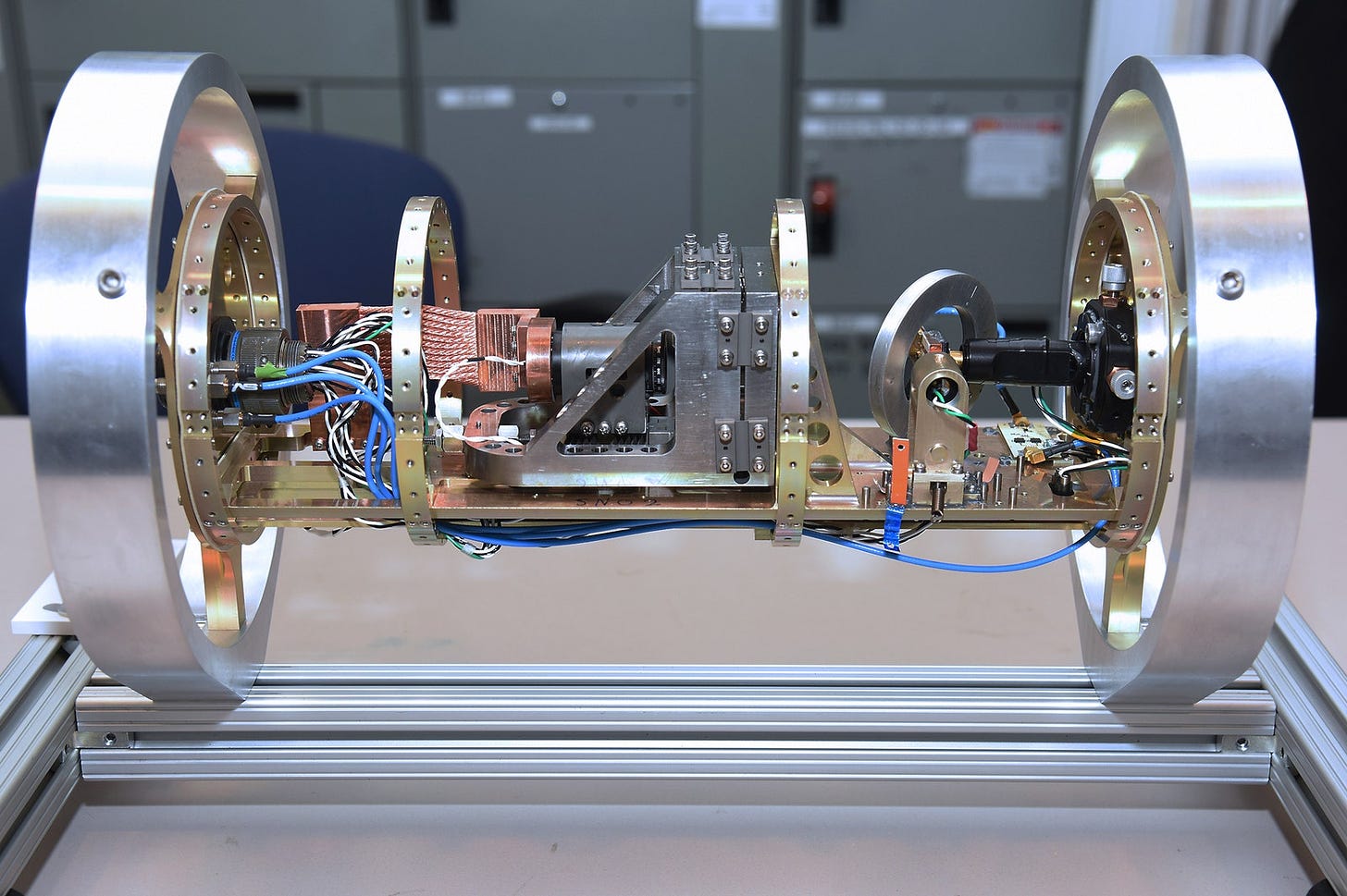
Lockheed Martin has already experimented with such a device although there is still more work to be done before we start seeing cars shipped with a quantum diamond GPS.
Conclusion
For thousands of years, humans have made tools to better understand the environment around them. The discovery of quantum mechanics has finally given us an understanding of nature at its smallest length scales and we now have the capacity to measure our environment to previously unthinkable levels of precision. With quantum sensors such as the SQUID and atom interferometers becoming mainstream, we must begin to consider the huge impacts they could have in the 21st century. From positive impacts such as safer buildings and reliable GPS to catastrophic ones such as greasing the skids of nuclear war, quantum sensing is rapidly evolving beyond the lab and is going to have huge impacts in the real world very soon.
For the sake of my own understanding, is the statement
"The answer is that gravity doesn’t affect light at all, but it does affect atoms."
just a simplification for the sake of the article? I was under the impression gravity *does* affect light by warping spacetime. Is this an "indirect" effect?
Not a quantum sensor, but neutrino detector (especially liquid Argon TPC types) being deployed for HEP have the ability to measure the neutrino spectrum from nuclear reactors from tens to hundreds of miles away.
Early (public) proof of principle experiments show the ability to detect when reactors are refueled or modified. So in principle, you can tell where another country's nuclear reactors are and what they are used for, in real-time, from another country.